How well can renewable energy meet Ireland's future needs? Feasta commissioned
the construction of a computer model of energy flows in the
Irish economy to find out. On one of the sets of assumptions set out here,
income growth will cease but CO2 emissions can be halved.
This paper describes a simulation study undertaken
with the aim of exploring the potential for
renewable energy to make a key difference to
the future sustainability of the Republic of
Ireland. We begin by looking at the electricity-generating
sector itself, and move out from
there to consider some complementary technologies
that will interact well with renewables-generated
electricity in moving Ireland towards
a more sustainable state.
The modelling approach we used was ECCO,
originally developed by Jane King & Malcolm
Slesser (Slesser & King 1993; Slesser et al,
1994, 1997). It provides a broad-brush sketch of
the entire economy which allowed us not only
to calculate the direct impacts of renewables on
the electricity-generating sector, but also the
synergies that may exist between renewable
generation of electricity and other technologies
and economic activities. We were not primarily
concerned with forecasting, but with representing
the potential for change of the economy
under a range of scenarios.
ECCO has a number of characteristic features,
physical, dynamic and holistic:
A) Physical
ECCO is primarily a physical account of the
economy. Energy analysis theory, as defined in
the proceedings of the IFIAS workshop of 1975
(IFIAS, 1975) underpins the model, and is
explained in the primer which follows this article.
It explicitly recognises the importance of
the second law of thermodynamics in limiting
the options available to the economy. According
to this law, any transformation to a system
incurs a net dissipation of energy and an overall
increase in the entropy (which can be thought of
as a measure of disorder) of the system.
Within a system, the entropy of a local
region can decrease if it is able to export the
entropy increase elsewhere. In industrialised
economies, we generally order our built environment
by exporting huge volumes of disorder
to natural ecosystems, as dissipation of energy
resources and as pollution. This behaviour was
first formally described in these terms by Ilya
Prigogine and colleagues as 'open systems'
(Prigogine & Stengers, 1984). National
economies such as the Republic of Ireland are
very much open systems, interacting not only
with natural environments, but with the global
political environment through trading goods,
services and financial flows. (Financial flows
have no direct physical presence themselves but
determine the direction in which physical effort
is expended).
By describing the economy in such physical
terms we get a direct handle on some of the key
interactions with nature, such as rates of fossil
fuel extraction, use of materials and emission of
atmospheric pollutants, as well as many interactions
that occur within the economy.
However, our concern with exact thermody-
namics does not overshadow our desire to accurately
describe aspects of the real world, and we
do not try to superimpose a grand theory of
energetics upon reality. Where appropriate,
other units are used to measure specific variables
(e.g. transport demand in passenger-km
and tonne-km for freight).
B) Dynamic
ECCO is a dynamic model. It does not describe
the state of the economy at a single point in
time, but the unfolding of events in the economy
over a period of decades. It is suited for
describing long-term economic patterns over
such timescales, but less suited for explaining
short-term fluctuations over periods of months
or quarters. The computational techniques used
to describe these dynamic relationships are
classical system dynamics, as developed by Jay
Forrester & colleagues in the 1960s at MIT
(Forrester 1968, 1971; Meadows et al, 1973).
The dynamic nature of the model is important
in deeper ways than simply allowing us to
describe key indicators as time series rather
than snapshot values. A simple linear programming
model can accomplish this. System
dynamics, however, excels at describing complicated
feedback interactions between many
factors, and the ECCO model contains many
feedback loops. These often lead to counterintuitive
behaviour in the model, that is, behaviour
that may seem to be unexpected at first
glance, but, when its causes are traced back
through the model structure, does make sense.
When we engage with the model in this way, we
are encountering questions about the way our
economy operates, and gaining insights that are
qualitative as much as they are quantitative.
As an example, a model may show that making
a large reduction in energy demand for a sectoral
activity at negligible capital cost causes
the economy's overall energy demand to
decrease initially, but increase in the longer
term. This latter increase is counterintuitive, but
can be explained by the mechanism that
decreased energy demand either benefits balance
of payments or reduces required domestic
energy extraction investments, allowing the
economy to grow at a faster rate than it otherwise
would. This 'rebound effect' is discussed in
greater detail in Slesser et al, 1997. The point is
that formalising the structure of the economy in
our model has brought to our attention an inherent
outcome of the assumptions that we have
fed into it that had previously not been noticed.
Even if we recognise that the model is a very
limited representation of reality, with many factors
simplified or omitted (and this is true of
any model, however detailed), we can take the
insight about rebound effects away with us and
consider its place in the real world. This qualitative
insight is arguably more important than
the numerical time series data that the model
generates.
The world-view of the dynamics modeller
informs our definition of sustainability too. We
do not seek to describe sustainability as an endpoint,
a goal that our model economy seeks and
then steps into some sort of steady state once it
reaches it. If we were to consider that an economy
achieved sustainability by 2050, and was
still sustainable in 2100, it is unlikely that the
two economies would be identical. They might
be radically different in some ways.
C) Holistic
ECCO is a holistic model. Rather than covering
one part of the economy in fine detail, the entire
economy is described in coarse detail. Specific
sectors may be developed to a greater level of
detail than others (in our case, electricity generation
and energy conservation, for example),
but all sectors are represented at some level of
detail. Because the model determines its own
growth rate (see below) it is important that we
know the demands and supplies associated with
all parts of the economy in order to assess the
overall growth potential. A sector described at
the lowest level of detail can be thought of as a
placeholder. It is unlikely to do anything surprising
during a simulation. When developing a
model for specific purposes, we need to evaluate
where we wish to apply detailed policy
options, and from there, what level of detail is
appropriate for the other major sectors. For
example, if we were to develop a model with
the aim of studying water usage, we would need
a high level of detail in the industrial, domestic,
agricultural and possibly electricity generation
sectors. Services and transport sectors could be
simpler, taking their cues from the ups and
downs of the more detailed sectors.
In some studies, the broad overview offered by
ECCO has been usefully combined with more
detailed static analysis (Crane & Foran, 2000).
Where this has been done, much of the crosstalk
between the models has been conducted
through a sharing of insights, rather than an
attempt to hitch the computational data streams
together into a single super-model.
SELF-DETERMINING GROWTH RATE
The model will determine its own growth rate
over time. Most dynamic economic models will
feed in the average growth rate as a user-defined
input. We allow the modelled economy to grow
as fast as is possible under the policy options
that are in place. User-defined policies may
well affect growth rates, albeit indirectly, and
these allow us to capture some of the more subtle
long-term effects of policy options (such as
the rebound effect described earlier).
The model's central 'growth loop' represents the
key influences described by the model that lead
to physical growth in an industrialised economy.
All variables here are referred to in embodied
energy terms. An 'industry' sector is defined
as containing all those activities that produce
physical goods. Other sectors are also defined,
such as agriculture, services, housing, etc.
These all contribute meaningfully to the economy,
and all require a stock of fixed capital
(buildings, machinery, etc.) through which to
do so. Only the industrial sector is able to supply
that capital - either the domestic industrial
sector, or one overseas, at any rate. International
trade complicates the picture a little, but can be
adequately handled by the 'growth loop' model.
As shown in Figure 3A1, the aggregated flow of
'human-made capital' (HMC) from the industrial
sector can be diverted to a number of purposes:
- consumption of non-fixed/disposable products
- reinvestment in and maintenance of the capital
stocks of industry
- reinvestment in and maintenance of the capital
stocks of other sectors
- exports of goods to other economies.
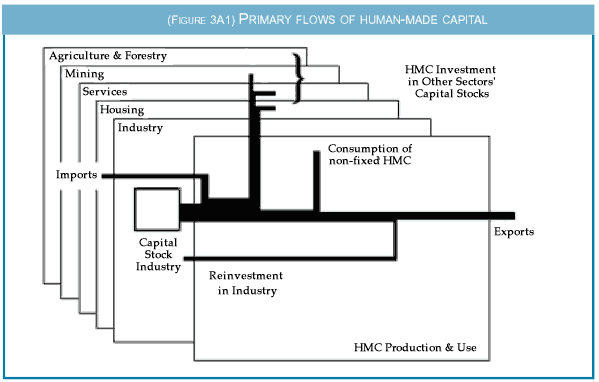 |
Human - made capital (HMC) produced by firms in the industrial sector flows to the other sectors of the Irish economy and is also exported. |
Note that all capital stocks depreciate (an
inevitable consequence of the second law of
thermodynamics, and a practical fact), and
maintaining a sector at a given size requires
continual investment. The overall demands for
reinvestment by all other sectors will be determined
by the assumptions we make about them,
and by policy options that we may set. For
example:
- required investment in energy will be driven
by market forces in most western economies.
Ultimately, this will depend upon a diverse
range of factors, such as energy costs of extracting
the fuels, comparative energy costs of alternative
fuels, and demands for the fuel by the
domestic economy and export market. All of
these factors can be represented in the model.
- required investment in housing will be driven
by population in a very simple model, and possibly
by a measure of affluence. In a more complex
model, we may also wish to introduce
average size of a household in capita terms.
Many western economies have seen considerable
decreases in average household size over
the last few decades, with considerable consequences
on per-capita consumption of energy
and resources of a domestic nature (a six-person
household does not generally own a house six
times the size of a one-person household, nor a
fridge or heating system consuming six times
the energy). If we choose to consider the social
dynamics of increasing divorce rates and
decreasing family sizes, we can see implications
for our physical growth loop.
We calibrate the rate of consumption of disposable
goods based on short-term indicators of
economic well-being, and, after factoring in
balance of payment adjustments, allocate the
remainder to industrial reinvestment. If this
amount exceeds the rate of depreciation in
industry, our industrial sector will grow over
time, and hence the future production of
human-made capital will increase, all other
things being equal (and we can break that
assumption and model effects of technological
change within industry if we wish to).
Conversely, if the reinvestment in industry is
too small, future output of HMC will contract,
to the detriment of the entire economy. Because
this reinvestment term is sensitive to changes in
consumption, in investment in every sector and
to international trade in goods, services and
financial flows, the growth of the model as a
whole is sensitive to a wide range of policy
options.
Figure 3A2 portrays the growth loop in terms of
influences between terms, with the arrows
pointed from influencer to influenced.
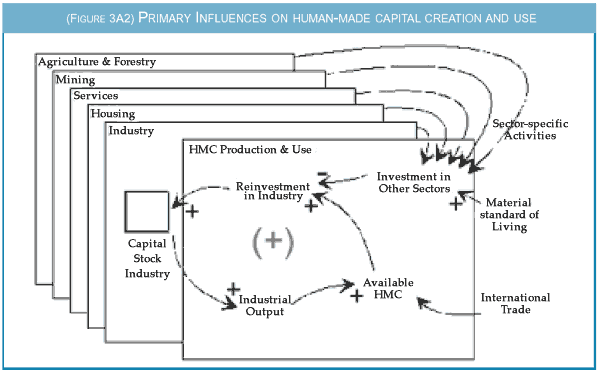 |
Feedback from the various sectors of the economy and from international trade influences the way that human-made capital (HMC) is allocated. |
A plus
sign indicates that the variables will move in the
same direction (as x goes up, it will push y up,
as x goes down, it will push y down), and a
minus sign opposite directions.
Finally, it is worth elaborating a little on the balance
of payments sector. In a national economy,
there will be significant trades with the outside
world, in physical goods, in services, and in
financial flows. The physical flows can easily
be incorporated into the above model, and in
some cases (notably energy resources) will
already be calculated by the model. In the case
of intangible trades, we convert these to a nominal
embodied energy value based upon the
average energy intensity of the economy, a term
that we calculate for internal accounting purposes
anyway. An economy that exports significant
intangibles (e.g. Switzerland) can, as a
result, afford a much greater inflow of HMC
than would otherwise be possible. The internal
costs of generating such flows can be accounted
for in our representation of the services sector,
which would probably be quite detailed for
such an economy.
THE IRISH MODEL
The pilot model of the Republic of Ireland was
developed over a period of approximately six
months. We aimed to replicate the broad growth
patterns of the Irish economy over the period
1990-2002 (or as recently as official time-series
have allowed), and then simulate them out to
2050. This was successfully achieved, although
inevitably some areas were sketched-in in relatively
little detail. In describing the model, it is
useful to note omissions as well as the detail
that has been included, particularly for the sake
of pointing to relationships between policies
that we may have failed to capture in this first
iteration of the model.
The model divides the economy into a number
of broad sectors, following the sectoral divisions
provided by the main data sources that we
used to calibrate it. These are:
- Agriculture, Forestry & Fishing
This sector was sketched-in in terms of fixed
capital stocks, and energy resource use. Future
growth is driven by domestic requirements and
export markets. No attempt was made to distinguish
between types of agriculture, nor to
assess land use. This latter would be required
were we to factor in the land-use requirements
of a large-scale biomass initiative (and, to a
lesser extent, land-based wind generators,
although it can be argued that these can happily
coexist with many types of crops).
- Mining, including gas and peat extraction
Our primary focus here was on describing the
reserves of natural gas and peat, and the fixed
capital stocks employed to extract them.
Investment in these sectors was driven by
domestic demand and world export markets
(significant only in the case of gas).
- Industry & Manufacturing
This sector was treated as a single entity,
although in reality it covers a range of activities
from heavy industry to food processing and the
construction industry. (Even in much larger,
more mature ECCO models we rarely disaggregate
this sector.) The effects of investment in
fuel-efficient technologies in this sector were
characterised.
- Utilities, primarily electricity generation
Electricity generation was divided into a number
of technologies, covering conventional fossil-
fired plant, combined-cycle plant (a more
modern technology with greater efficiency of
use of fuel), conventional hydro-electricity, and
renewables. Separate subsectors were drawn up
to characterise wind generation, photovoltaics,
biomass-generated ethanol and methanol plant,
and wave energy, although our analyses here
focussed primarily on wind power. Userdefined
policies for future uptake between
existing technologies were developed, with
Business-As-Usual policy allocating 90% of
new plant built beyond 2000 to combined-cycle
fossil plant, and 10% to renewables. The differences
between technologies were characterised
primarily as:
- Load Factor i.e. percentage of time a plant
would typically be online
- Thermal efficiency of converting fuel to
electricity (where the plant is fuel-powered)
- Fixed capital cost of building and maintaining
plant
We did not account for one potential benefit of
wind power, that the technology could be developed
indigenously, and potentially sold worldwide,
whereas fossil-fired futures would be
dependent upon foreign technology bases (and
therefore represent a poorer situation for international
balance of payments).
- Services
The services sector was characterised in terms
of fixed capital and physical resource consumption
(e.g. thermal fuels, electricity). Demand for
services was limited to the three major consumer
sectors, industry, domestic dwellings,
and the services sector itself.
- Domestic dwellings
These again were characterised in terms of
fixed capital and resource requirements. Effects
of domestic energy efficiency technologies
were taken into account (and here the payback
could be significantly greater than in the industrial
sector, owing to the poor starting position
of the Irish housing stock in terms of simple
energy efficiency 'quick wins' such as insulation),
as well as potential for electricity-powered
ground source heat pump technologies.
- Transport
The transport sector does not maintain its own
capital stock, as national accounts data
describes the fixed capital in transport machinery as belonging to the owning sector e.g.
industry, services, domestic. Energy use by
transport is defined separately, though, and we
were able to calculate demand based on passenger
and freight volumes. Policies describing
potential future modal splits between transport
sectors are in place, as well as future fuel mixes,
including electrically-powered passenger vehicles
utilising fuel cell technologies.
- International Finance
This sector defines the net impact upon the
growth of the economy of international trade.
Some parts of this can be characterised well by
the model, such as the necessity of fuel imports,
others are defined as user policies, such as
future levels of international investment capital.
Over the calibration period, Ireland enjoyed an
unusually high influx of international capital,
which we have allowed to decrease somewhat
in the Business-As-Usual profile for the model.
DEFINITION OF SUSTAINABILITY
Sustainability is an ill-defined and much-abused
term. For the purposes of assessing the
relative merits of the outcomes generated by
our scenarios, it is useful to have one or more
sustainability indicators to hand. For this study,
we focus primarily on energy-based measures,
because we are looking at energy-related scenarios.
Carbon-dioxide emissions are a good sustainability
indicator, and can be readily compared
against the agreed Kyoto targets for the Irish
Republic. If we define energy sustainability as
receiving all energy requirements from renewable
resources, we can compare our progress
towards that goal by plotting fractions of energy
derived from renewables, both for electrical
energy and all primary energy. We also created
a third variant for this study, in which we
included indirect imports of primary energy in
an attempt to reflect the energy expended elsewhere
in the world. This would counter the
'accounting loophole' of the simpler indicator
whereby an economy could simply shift energy-
intensive activities overseas. In the case of
the Irish model, it made only a slight difference
in most cases.
In terms of security of energy supply, we could
compare Irish demand for exported oil and gas
against projected world outputs of oil and gas
(using data from Campbell, 2002). Again, it is
worth stressing that our assessment of the sustainability
of each outcome does not end with
the set of indicators outlined above, and that it
is necessary to step back from the model and
consider the results in the light of the real
world.
CALIBRATING THE MODEL
The model was initialised for the year 1990,
and run over a ten-year period against real historical
data in order to calibrate it. The primary
data sources used to calibrate the model were:
- National accounts data from the Central
Statistics Office of Ireland (www.eirestat.cso.ie), particularly for the purpose of getting
Gross Domestic Fixed Capital Formation
(GDFCF) data (series naaa04xx) upon which to
base the model rates of capital formation. In
this case, as no Fixed Capital Stock data was
available from the CSO, the GDFCF data was
also used to estimate initial capital stocks by
using a simple spreadsheet model that tracked
growth and depreciation of sectoral capital
stocks over the calibration period. The CSO
bpaa series data was also used to calibrate the
international finance sector of the model.
- Energy balance data for Ireland, from the
Department of Public Enterprise. The report
"Energy in Ireland" provides data for the period
1980-1993. Energy balances for 1994-2000
were taken from the DPI website
(www.irl.gov.ie/tec/energy/statistics ).
These provide a detailed breakdown of energy
resource production, imports and domestic
usage by sector, as well as transformations into
secondary fuels such as briquettes and electricity.
Combining the energy balances over the
calibration period, it was possible to develop
detailed time-series data of sectoral energy
usage by fuel type.
- The Electricity Supply Board Annual statements
contained much detailed information on
generating capacities and types of generator,
from which a more detailed description of the
electricity generation sector could be developed.
- The final report of the EU-funded ALTENER
report "Total Renewable Energy Resource in
Ireland" provided helpful information when
developing the renewable energy sector.
This calibration process led to the definition of
a business-as-usual (hereafter BAU) scenario,
in which current technologies and policies were
extrapolated out over a further fifty-year period.
This is not intended to provide for an accurate
forecast of Ireland's future, but to develop a
well-defined baseline against which we can
compare the effects of the changes we introduce
in our policy studies. In the electricity-generating
sector, we assumed that the majority
of new generating capacity would be gas-fired,
with a small fraction (10%) being wind energy.
We assumed a continued high level of investment
in Ireland from overseas, although less
than was seen in the 1990s, recognising this to
be an unusual decade. Key assumptions of the
business-as-usual scenario defined for the purposes
of this report are outlined in Figure 3A3.
Key features of the outcome of the Business-
Scenario Policy Inputs / Scenario Definition
As-Usual scenario are outlined below:
- The economy continues to grow, but very
slowly (figure 3A4).
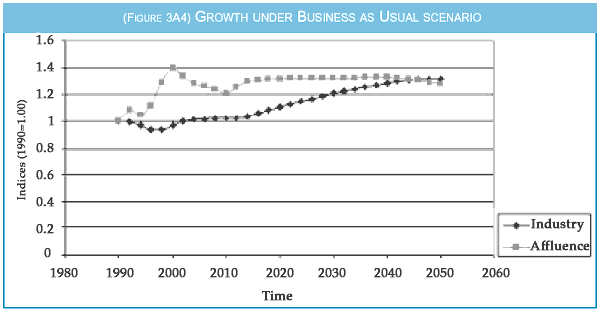 |
Growth Indicators under BAU scenario. Growth in both personal material affluence and manufacturing capacity is slow |
Our key indices of growth,
for average per capita material affluence and
manufacturing output, show less than a 50%
increase over the 50 year period, equating to an
average growth rate of less than 1%. This slow
growth is in spite of the continued inward
investment from overseas, and partly reflects
the longer-term effect of this, as returns on the
investment begin to leave the country.
- Carbon dioxide emissions show a decrease
from through to 2030 (figure 3A5), owing to a
combination of structural changes and economic
decline.
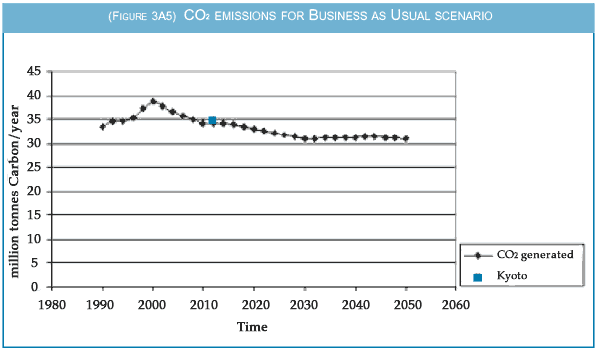 |
Projected CO2 emissions under Business as Usual. The temporary decline in emission rates (which does not mean a decrease
in atmospheric CO2 levels) can be explained by looking at the changing structure of the electricity generating sector. Note that
the model underestimates CO2 emissions for 2000 by roughly 5%, as the actual figure was around 41 million tonnes
carbon/year. |
Beyond 2030 they remain essentially
flat, in step with the negligible economic
growth rates during the latter phases of simulation.
The decrease from 2000-2030 can be
explained by the phasing out of the current
cohort of conventional fossil-fired electricity
plant, and its replacement by more efficient
combined cycle plant (figure 3A6).
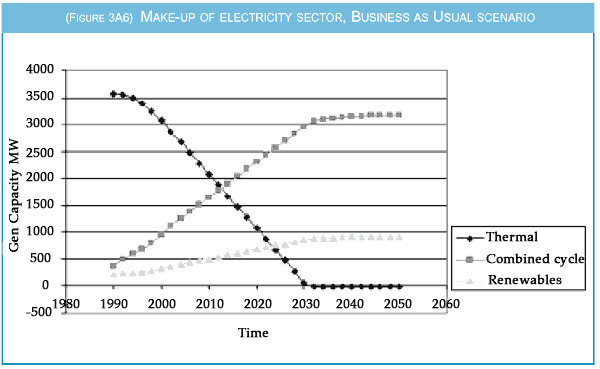 |
Make-up of electricity sector generating capacity under Business as Usual. Existing thermal capacity declines through to 2030,
and the majority of new capacity is introduced as combined-cycle plant. Renewables play a minor but not
inconsiderable role. |
This in
itself is enough to meet the Kyoto requirements
for 2012, with no positive action required. In
terms of energy policy, it is apparent that there
is a window of opportunity over the next thirty
years within which alternative energy strategies
can be developed while enjoying the benefits of
the decline of this technology. Beyond 2030,
achieving an active reduction in CO2 emissions
would not be so easy, particularly if other policies
were brought into play during the next fifty
years that resulted in positive economic growth.
The lukewarm 'good news' of matching the
Kyoto targets shown here would be better characterised
as a combination of opportunism and
economic doldrums, and shouldn't be mistaken
for actively getting to grips with the required
decoupling between economic activity and fossil
fuel use. (It is also worth pointing out that a
flat CO2 profile is not particularly good news
for the environment, as it simply represents a
steadily increasing stock of CO2 in the atmosphere
rather than a rapidly increasing one.)
- Dependency on imported fuels remains very
high. Native supplies of natural gas are already
mostly exhausted apart from the recently discovered
Corrib field off the coast of County
Mayo. We decided to treat this field as a nonnative
resource as its owner, Shell, will find the
capital for its exploitation outside Ireland and
send almost all the money it receives for the gas
outside Ireland too. As this makes gas from
Corrib functionally identical to gas coming into
Ireland across the interconnector, it allows it to
be left out of the model. Ireland's only other
indigenous fuel, peat is a small fraction of the
total energy demand. Major fuel demands
include natural gas for electricity (21% of total
fuel demand by 2050), oil fuels for the transport
sector (42% of total fuel demand by 2050) and
domestic heating fuel (18% of total fuel
demand by 2050). In terms of fuel supply security,
the combination of declining native gas
supplies and buying into a gas-dependent electricity
future do not make for a good combination.
SIMULATION EXPERIMENTS
A number of simulation experiments were conducted
to assess the viability of some proposed
solutions to Ireland's (and the Western world in
general's) current sustainability problems of
reliance of depletable resources and increasing
carbon dioxide output. The options assessed
here are primarily technological. Figure 3A7
contains a brief summary of the changes to the
BAU profile made in defining all the scenarios
undertaken in this report.
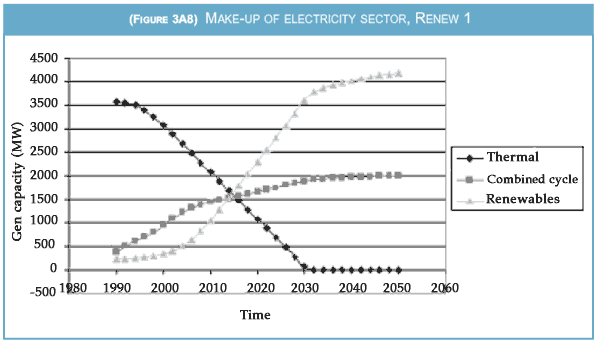 |
Make-up of electricity generating capacity under first renewables scenario: renewables rapidly ovetake CC technologies to
become the dominant technology. |
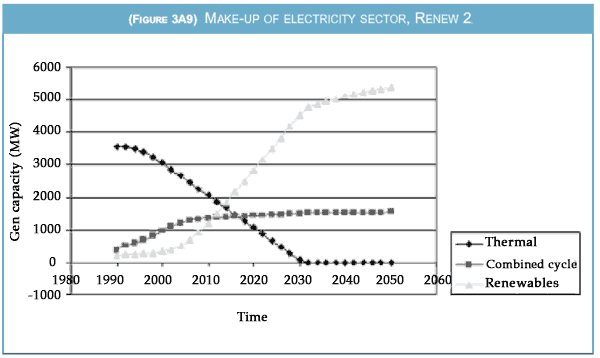 |
Under the second renewables scenario, the situation is similar to Figure 3A8, only somewhat more rapid. |
RENEWABLE ELECTRICITY
Ireland has significant potential for renewable
electricity generation, primarily in the form of
wind and wave. Bio-fuels are also an option,
although not one that we examined in detail
here, as doing so would require a more detailed
model of land-use interactions between fuel
crops and other forms of agriculture than we
have currently developed. Compared to gasfired
combined cycle plant, wind power is
roughly 3 times more expensive to build (the
capital costs per megawatt of generating capacity
are not widely divergent, but the load factor
for CCGT is much higher, leading to a far
greater return in terms of electricity generated).
Potentially, a large-scale adoption of wind
power would have a negative impact on economic
growth as a result of this extra capital
expenditure (in the BAU scenario, investment
in the electricity sector accounted for roughly
5% of total investment in fixed capital stocks).
Although the economy can support the more
extreme penetration of wind power, overall, this
has a relatively minor impact on the sustainability
of the nation. As we pointed out earlier,
there are major direct demands for fossil fuels
outside of the electricity sector. Figure 3A10
plots the rather smaller share that renewable
electricity gains of the economy's total energy
usage.
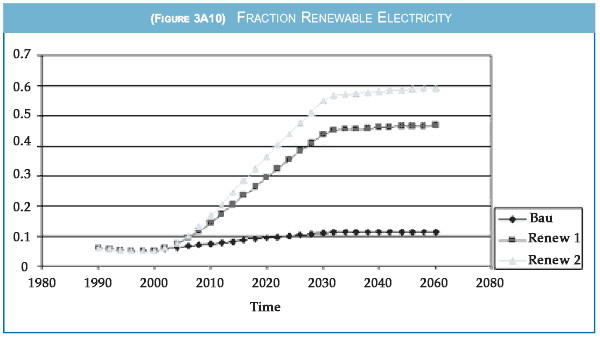 |
Fractions of electricity generated by renewable resources under the three scenarios |
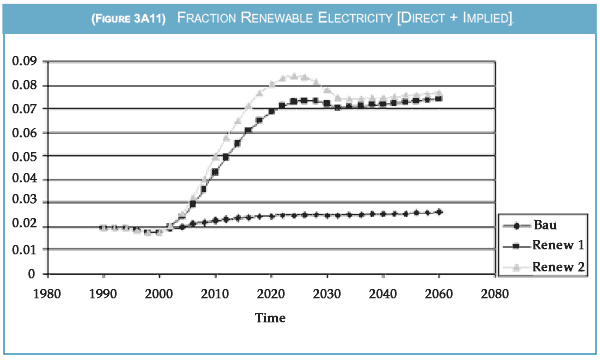 |
Fractions of electricity generated by renewable resources under the three scenarios |
The 9-10% share achieved by the second
renewables scenario is certainly an improvement
on current arrangements, but is unlikely to
make a long-term difference environmentally
(the CO2 profile for this scenario is only 5%
lower than for the BAU scenario by 2050). If
renewables-generated electricity is to have a
serious impact, it must also begin to replace
some of these other demands. The remaining
scenarios look at some options for doing this.
RENEWABLES-POWERED TRANSPORT
Fuel cells represent one technology that may be
used in the transportation sector as an alternative
to continued fossil fuel dependency. We
made a broad assumption that an electricitypowered
car could convert energy to motive
power with roughly twice the efficiency of a
petrol engine. The conversion of fuel to electricity
(the thermal efficiency) for conventional
electricity generators is roughly 30-35%, with
combined cycle plant achieving a somewhat
higher value. Hence, from a system-wide perspective,
the use of fossil-fired electricity to
power transport makes little sense.
In the case of renewable electricity, of course,
there is no initial fossil fuel input, and a clear
case for sustainable transport through use of
fuel cells can be made. We adopted the more
extreme renewable energy policy at the same
time as driving a significant substitution of conventional
road transport with electrical vehicles,
achieving 50% penetration by 2030 and a
75% penetration by 2040. This has a significant
effect on the demand for thermal fuels by transport,
as shown in Figure 3A12, and reduces the
total energy demand of the economy by around
7% by 2050 when applied by itself, and has a
marginally positive effect on the fraction of
renewable energy use indicator (Figure 3A13).
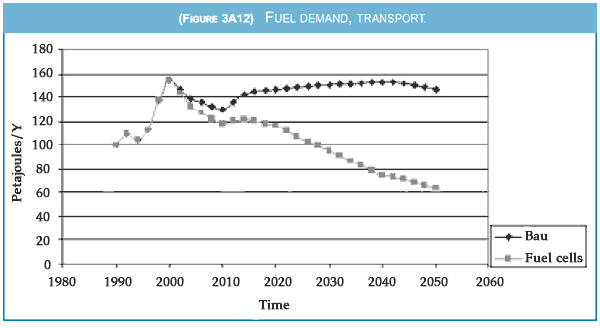 |
Fossil fuel demand by transport under Business as Usual and fuel cell scenarios |
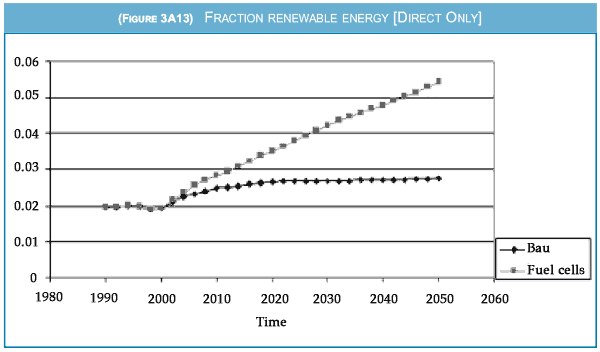 |
Fractions of energy supplied by renewable resources under Business as Usual and fuel cell scenarios. |
Domestic energy conservation
Ireland's housing stock generates a significant
thermal energy demand, used almost entirely
for space heating. The current housing stock
can be characterised as poorly insulated, with
even modern dwellings tending to opt only for
simple cavity insulation. A number of quick
wins could be made by insulating this housing
stock. We characterise the impact of investment
in domestic energy efficiency as one of diminishing
returns, assuming that the first investments
made are the most cost-effective. The
data we use is based on a study by the TNO,
Netherlands (Melman et al., 1990), and probably
under-represents the capacity of the Irish
housing stock to benefit from such investments.
Nonetheless, with sufficient investment, energy
savings of up to 50% would be feasible,
although this would require significant additional
investment.
Under the domestic energy scenario, we invest
additional resources in the housing stock with
the express purpose of applying energy conservation
technologies, matching the main investment
in the domestic sector by 5% in 2010
through to 20% by 2030 onwards. The knock-on
effect of this is to reduce energy consumption
per household by 20% by 2050, as these
investments trickle through the existing and
new housing stocks (Figure 3A14). Overall
effects on the fossil fuel dependency of the
economy are very marginal (Figure 3A15).
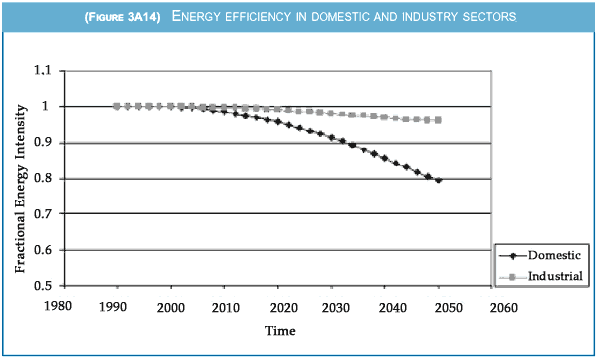 |
Energy savings arising from domestic energy efficiency investment |
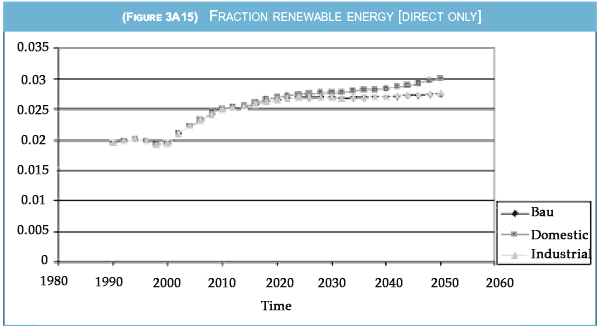 |
Domestic energy efficiency policy has a minor impact on the fraction of national energy demand met by renewables. |
Industrial energy conservation
Industry and manufacturing is also a large consumer
of fossil fuels. Here, the fuels are used to
provide a mixture of heating and motive power,
as well as cogeneration of electricity. Following
the TNO study data, we assume that the returns
on energy efficiency investment in manufacturing
would be less than with the housing sector,
with a maximum reduction of around 10%
being achievable. These figures are, again,
probably rather cautious, but we present them
here in the absence of any other hard data.
The scenario here was defined in an identical
fashion to the domestic energy efficiency case,
with a ramping up of additional investment to
match 20% of the primary investment in the
sector by 2030. Figure 3A14 shows that the
reduction per unit of operational stock is much
less than for the domestic sector, and Figure
3A15 confirms that the impact on the national
renewables balance is negligible.
Domestic heat pump technologies
An alternative (or complement) to domestic
energy efficiency is the use of electrical heating
for homes. This makes very little sense when
electricity is generated by fossil fuels (up to two
thirds of the heat content of the fuels goes up
the generator chimney and one third is delivered
to the home), but under a predominately
renewable energy regime it might make more
sense. Rather than looking at systems that convert
electrical energy directly into heat, however,
we chose to examine heat pump technologies,
in which the heat energy generated is
extracted from ambient heat gradients in the
ground, and the electricity is simply used to
access this rather than being the primary source
of the heat.
In characterising the technology, we assumed
an average thermal efficiency of traditional
domestic heating of 80%, and a coefficient of
performance of the heat pumps of 25% (i.e. 4
units of electricity, measured in heat energy
terms, are required to transfer one unit of heat
energy). The example scenario pushes the technology
quite aggressively, aiming at 10% penetration
of the housing stock by 2010, through to
a 50% penetration by 2050. Predictably, this
roughly halves the domestic sector intake of
fossil fuels (Figure 3A16), and increases electricity
demand by dwellings significantly (but
not by 50%, as a large fraction of this is
required to power consumer goods of various
sorts as well) - (Figure 3A17).
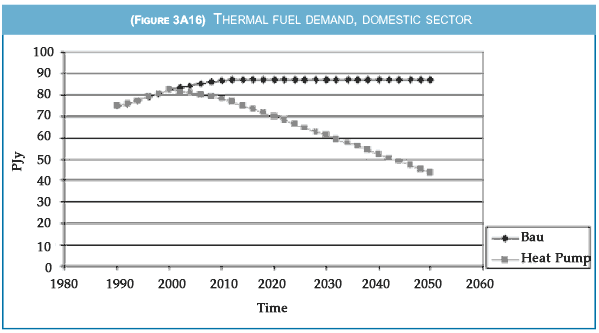 |
Thermal energy demand by dwellings under Business as Usual and domestic heat pump scenarios. |
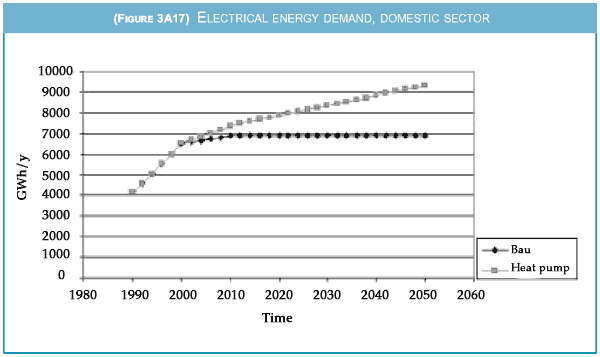 |
Electrical energy demand by dwellings under Business as Usual and domestic heat pump scenarios. |
Again, we see a
mild improvement in the national renewable
energy balance (Figure 3A18), and this leads to an
increase in total renewable electricity generating
capacity of 10%.
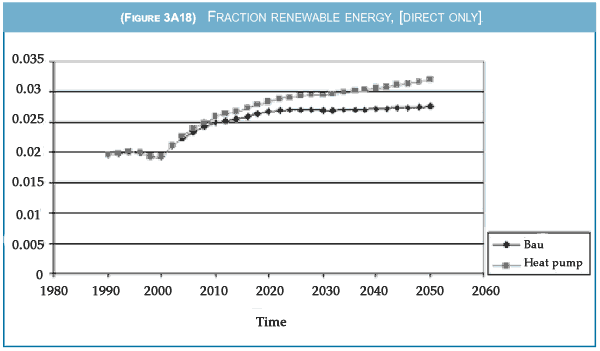 |
Fraction of national energy demand met by renewables under Back At Work and domestic heat pump scenarios. |
All of the above
Finally, we adopted all of the above measures at
once.
- A large-scale substitution of renewable electricity
technologies (wind-powered), with 80%
of all new generating capacity being wind.
- Large-scale transition towards electric fuel-cell
road transport
- Significant investment in domestic and industrial
energy conservation
- Widespread adoption of heat pump technologies
for domestic heating
As outlined in Figure 3A8, we introduced these
both at the magnitudes already examined oneby-
one, and then finally at a greater rate, simply
in order to see how far we could achieve sustainability
by the measures we had focussed
upon.
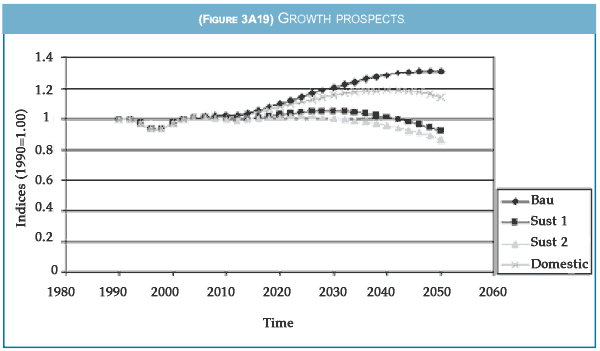 |
Sum effect of sustainability policies on growth |
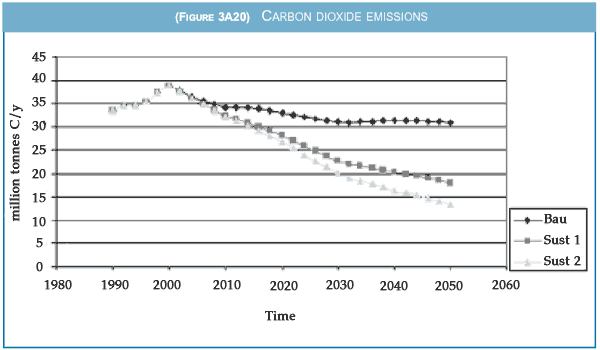 |
Sum effect of sustainability policies on CO2 emissions |
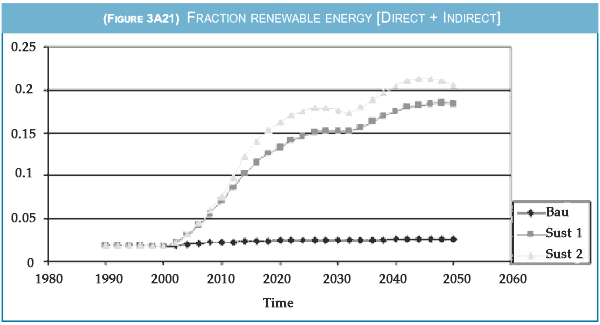 |
Fraction of total energy demand met by renewables under combined sustainability scenario |
CONCLUSIONS
The Republic of Ireland is capable of making a
transition towards sustainability over the next
fifty years, owing to a plentiful supply of
renewable resources and modest growth
prospects. Structural factors such as the decline
of current 'dirty' generating technologies, and
the quick wins available from a poorly insulated
housing stock, also serve to play a part.
While renewable electricity generation can play
a major part in this transition, a simple
approach aimed only at replacing current fossil-fuel
generation of electricity with renewables
will have a limited effect. A much greater effect
can be had by looking for synergies with other
parts of the economy, where electricity-based
technologies that would simply be inefficient
(in system-wide energy terms) can be brought
into play. The more high-tech options considered
here, such as car fuel cells and heat transfer pumps, are wasteful of fossil fuel if fed on
fossil-generated electricity alone, but can
become very useful in widening the circle of
influence of a renewable electricity technology.
The renewable electricity industry would do
well to seek partnerships with developers of
such technologies, in our opinion.
The technologies that we have looked at in this
study are by no means exhaustive, and a more
thorough cataloguing could doubtless achieve
even greater gains towards sustainability than
we managed in our final scenario. There are
other renewable energy technologies, such as
wave power, solar-power systems (perhaps less
of an opportunity in the Irish climate, but still
offering some potential) and biofuels, which can
be represented by the ECCO methodology (and
have been in other studies). There are many
other technologies that may substitute fossil fuel
consumption for electricity use, developing further
synergies with an expanding renewables
sector.
As with any modelling study, we have presented
a greatly simplified picture of a real economy,
and left out much detail. In balance of payments
terms, the economy may benefit from technical
leadership in renewable technologies, which it is
far more likely to develop through early adoption
than in the already-saturated market for
combined cycle turbines. We have not examined
in detail the land-use implications of our policies,
what the spread of wind generators across
the landscape would look like (and, were we to
consider biofuels, this would be an even more
important aspect to factor in). We have not discussed
nuclear power as an option here,
although the model is capable of representing
the shorter-term costs and benefits of that technology
at least.
This is one of almost 50
chapters and articles in the 336-page large format book, Before the Wells
Run Dry. Copies of the book are available for £9.95 from Green Books. |
 |
Continue to Energy Analysis: a primer
Sitemap for Before the Wells Run Dry